Copper's Role in the Safe Disposal of Radioactive Waste: Copper's Relevant Properties - Part I
Copper Applications in Health & Environment
Introduction
When it comes to nuclear energy, people tend to be staunchly in favor (Clean energy! Reduce strip mining! Cut dependence on foreign oil! No greenhouse gases! Reduce global warming!) or passionately opposed (Dangerous radioactivity! More Chernobyls! Increase cancer! Proliferate plutonium! No more nukes!) But whether they're pro or con, most folks agree that disposal of the so-called "nuclear waste" produced by nuclear power plants presents a serious and global environmental problem.
Keeping radioactive waste (or "radwaste") out of the environment is clearly a very serious issue. It is, quite rightly, the subject of intense research and development in many of the 32 countries that operate the world's 442 or so commercial nuclear power plants. (The U.S. currently has 109 operating nukes, which produce about 14% of the nation's electric power.) The technical crux of the problem is to find ways to isolate the waste safely for the several hundred thousand years it will take for the wastes' radioactivity to have decayed to near background levels.
Most of the currently proposed disposal programs rely at least in part on sturdy burial containers made from materials that have high corrosion resistance. Copper will play an important role in several of these programs. Copper won't be the universal solution - there are very sound reasons why it can't be used everywhere - but in at least two European countries so far, copper will be a key barrier standing between the waste and the biosphere.
In this article and others to follow in the coming months, Innovations will describe copper's role in radioactive waste disposal. We'll describe how copper's properties make it attractive to disposal technology; we'll examine why copper was considered for the U.S. and Canadian disposal programs (and why it wasn't selected), and we'll describe the disposal systems in which copper will be utilized.
But first, a quick primer on radwaste to explain what this is all about.
What is radioactive waste?
Officially, "radioactive waste" refers to any no longer useful material that is itself radioactive or is contaminated with radioactive debris. It's a very broad term, and the level of radioactivity needn't be very high for something to be considered radwaste. In terms of sheer volume, most radwaste consists of slightly radioactive leftovers and contaminated trash from one of the many nuclear related technologies, like, for example, the manufacture of household smoke detectors.
Such material is classified as Low Level Waste (LLW). LLW is compacted, sealed in containers and shipped to disposal sites located around the country. Aluminum bronze, a strong copper alloy, has been recommended for containers to isolate the more highly radioactive forms of LLW (including what is termed "Greater-Than-Class-C Waste"), but steel, plastic and concrete are currently preferred.
On the other end of the spectrum is the waste that is so radioactive it must be handled remotely because even momentary exposure could be fatal. Usually, this also includes the waste whose radioactivity decays slowly, requiring that the material be isolated for long periods of time. It is here that copper will play its important role. The dangerous forms of radioactive waste include (according to U.S. Nuclear Regulatory Commission (NRC) definitions):
- High Level Waste (HLW) that results from the reprocessing of spent reactor fuel, plus similar materials that are overseen by the U.S. Nuclear Regulatory Commission (NRC);
- Defense High-Level Waste (DHLW) from weapons production and other military uses;
- Commercial Spent Fuel (CSF) and so-called DOE Spent Fuel. The latter comes from naval propulsion reactors, plutonium-production reactors, research reactors and other reactors operated by the U.S. Department of Energy (DOE).
Roughly one-half of the US's radwaste is CSF, the burned-out fuel from our nuclear power plants. Some of the fuel is more than 30 years old, but it is still very radioactive.
The U.S. is one of several nations that will bury its CSF intact, i.e., without reprocessing. In other words, the fuel will remain in its fuel assemblies. Many nations, including the UK, France, Germany, India, China and the former Soviet states, among others, are reprocessing their spent fuel in order to recycle and re-use the plutonium and uranium it contains. Besides uranium and plutonium, reprocessing generates a lot of unused radioactive material, some of which is dissolved in a strong vitreous material called boro-silicate glass. The glass is cast into large stainless steel bottles to prepare it for disposal. Since the by-products of fuel reprocessing are highly radioactive and remain so for a long time, countries that reprocess their fuel will also construct underground waste repositories.
How Much Waste is There?
US commercial power reactors currently discharge about 2,000 metric tons of spent fuel per year. To date, we have accumulated more than 30,000 MTU in the form of more than 100,000 spent fuel assemblies 1 , plus about 400,000 cubic meters of HLW. 2 All but about 750 tons of the country's CSF is stored in special water filled storage pools at nuclear power stations; the remainder is held above-ground in massive steel and concrete containers located that three storage facilities. In terms of volume, the spent fuel discharged by US reactors between 1968 and 1994 amounts to 14,091 cubic meters (476,394 cubic feet) or about the amount that could be piled onto an acre of ground to a height of eleven feet.
Recent estimates (see 2 and 3 ) place the total amount of spent fuel to be generated by US power plants by 2030 between 84,100 and 87,000 MTU contained in 293,000 to 299,000 fuel assemblies. The assemblies will occupy a volume between 37,800 and 38,600 cubic meters (1.3 and 1.36 million cubic feet). The one-acre pile would then be more than 30 feet high.
According to the American Institute of Physics 4 , the US's CSF inventory accounts for about 18.3% of the spent fuel stored worldwide; The UK (16.6%), Canada (15.4%), France (14.9%) and the former USSR (9.9%) also hold large quantities. In short, there will be a lot of radwaste to bury.
What makes copper an attractive candidate for radwaste disposal?
Before high level radioactive waste (including spent reactor fuel) can be buried, it will be encased in one or more containers. 5 The design of these containers are not fixed yet because the properties of the containers must be compatible with the repository environment, and most countries' repository sites won't be officially designated for several years. Still, prototype burial containers have been the subject of extensive research and development programs in several countries, including the US. There are at least as many proosed designs for containers as there are proposed repositories.
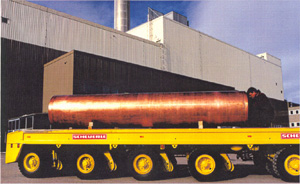
The containers are only one barrier against the release of radioactive species. Under today's commonly accepted multi-barrier disposal concepts, other barriers include such things as the fuel itself; the fuel rods' zirconium alloy cladding; the burial container(s); backfill surrounding the container, and the hundreds of feet of rock between the repository and the surface. Still, many disposal schemes require that the container be durable enough on its own to prevent the release of radioactive materials for long periods of time. One plan considers the spent fuel to be benign once it has reached the level of radioactivity from an equivalent amount of uranium ore from which the fuel was initially produced. For typical spent fuel, that would require about 240,000 years. Curves for several types of spent fuel can be found in one of the Department of Energy's web sites. 6
Corrosion resistance therefore becomes one of the waste container's principal requirements. From the standpoint of selecting a container material, scientists have three choices:
- Materials that are inert to all foreseen environments;
- Materials that form protective surface films that retard or inhibit corrosion, and
- Metals that corrode at a predictable rate and can therefore be made thick enough to last for the expected life of the repository. Such metals are known as "corrosion allowance" materials.
When it comes to corrosion resistance, the inert noble metals - gold, silver, platinum and copper (yes, copper is chemically classified as a noble metal) - top the list. They remain stable under well understood conditions. Among these, copper is the only practical choice.
Film forming or passive metals include titanium and its alloys, stainless steels and, under certain chemical conditions, copper and copper alloys. Several countries, including the U.S. and Canada, are considering passive metals for their disposal containers. The inherent difficulty with the passive metal approach, however, is that is assumes that the environment which gives rise to the passive film will not vary over time. There are other potential problems, as well. Some passive metals (titanium, for example) are difficult to fabricate and others are prone to cracking under certain environments.
Corrosion allowance materials are also viable candidates for radwaste containers, and several countries seem inclined to use them. Under certain conditions, the corrosion rate of copper and copper alloys is also highly predictable; so copper-base materials are also candidates under this containment scenario. The principal shortcoming of reliance on corrosion allowance materials is high weight due to the extra metal required to ensure sufficient life. Very heavy containers might present handling problems.
Because repositories are very conservatively designed, containers are generally expected to prevent release of radioactive agents for a portion of the total repository life time, and that portion might be shorter than the containers will actually last. Credits for the remaining time are allocated to the fuel, backfill and geologic structure. Designs vary widely among the many disposal programs now under development. For example, the US program credits its proposed stainless steel/nickel alloy container with a lifetime of 10,000 years, while the Swedish disposal program's outer copper container is expected to isolate the spent fuel for 100,000 years.
There is abundant evidence for copper's excellent corrosion resistance. Copper plumbing tubes operate trouble free for decades; ancient copper coins remain intact after centuries in shipwrecks, archaeologists find copper artifacts that are at least 8,000 years old. That's certainly long life, but it's not nearly long enough for radwaste burial containers. Under the right geologic conditions, metallic copper can resist corrosion 2000 to 3000 times longer than any repository is expected to survive. Many examples of this can be found in nature; some of the best are in the Upper Peninsula of Michigan, a former copper mining district. We'll say more about this "natural analog" repository in a future Innovations article. Toughness is another important requirement for radwaste burial containers. Containers will have to be rugged enough to withstand the stresses of manufacture, loading, handling and transport to the repository without damage. They should also be ductile enough to avoid rupture in the event a container is accidentally dropped.
Copper is a very ductile metal. It is easily worked into thin sheets and fine wires. Copper remains ductile even at low temperatures, and it can tolerate impact type loads without shattering. (Low temperatures are unlikely early in a repository's life, but repository planners think long-term, and the possibility of a future Ice Age cannot be discounted.) Strength, somewhat surprisingly, is not an absolutely crucial requirement. It is a relatively simple matter to engineer the needed strength into a container by providing reinforcement, using multiple vessels (nested inside one another) and other techniques. A future article in this series will describe how copper's moderate strength (and high corrosion resistance) is accommodated in multi-layer containers being tested for use in Sweden and Finland.
On the other hand, fabricability is very important since large numbers of containers will be required. In the U.S. alone, it has been estimated that about 19,800 burial containers will be produced through 2030 just to accommodate high-level wastes. 7 Several tens of thousands more will be needed for spent fuel assemblies and other high-level wastes. Up to four times as many containers will be needed for the rest of the world's radwaste.
While safety is obviously the primary consideration, cost cannot be ignored. The relative ease with which large copper can be fabricated presents definite economic advantages. Copper is routinely cast, rolled and forged into a wide variety of large complex articles. It is readily weldable; in fact, studies have shown that a technique known as electron beam welding can reliably seal thick-walled copper containers without over-heating the spent fuel inside.
Copper has what it takes
A number of copper's properties make it an attractive candidate for radioactive waste burial containers. Given the proper natural environment - and there are many that will do adequately - copper would last virtually forever without corroding, cracking or otherwise permitting radioactive release from a repository into the environment.
In the next article in this series, Innovations will look at the huge US and Canadian radioactive waste disposal programs to see where and why copper was seriously considered as one of the principal container candidates. Future articles will look at the Swedish and Finnish disposal programs, in which copper is currently accepted as the container material of choice.
References:
1.A fuel assembly contains either 63 or 264 fuel rods, depending on reactor type.
2.The data can be a bit confusing here. The DOE reports CSF inventories in units of metric tons of contained uranium (MTU) or, when considering burnup, metric tons of initial heavy metal (MTIHM). The given weight doesn't count fission products and light elements present in the fuel or the fuel's cladding and other metal structural members that make up the fuel assembly. The actual weight of accumulated CSF therefore exceeds 30,000 metric tons. For more information see a description of the US radioactive waste management system at U.S. DOE
3. Ibid
4. American Institute of Physics
5.Technical literature and government reports also refer to burial containers as canisters, overpacks and "engineered barriers".
6. U.S. DOE
7. Ibid
Also in this Issue:
- Alloy 171 for Electronic Interconnects
- Copper's Role in the Safe Disposal of Radioactive Waste: Copper's Relevant Properties - Part I
- Q & A With Hans-Erhard Reiter of the ADSL Forum
- Copper and Aquatic Life
- Research on Copper Joining Techniques Evaluates New Designs and Brazing Methods