Phosphor Bronze: Teaching an Old Dog New Tricks
Copper Applications in Metallurgy of Copper & Copper Alloys
Introduction
Just when you think a material has been developed as fully as possible, someone comes along and proves you wrong. The material: phosphor bronze; the someone: Olin Corporation.
Phosphor bronzes have been around for a long time. In strip form, they are widely used for, among other things, electrical and electronic connectors. The alloys typically contain up to 10% tin and up to 0.35% phosphorus. (All compositions mentioned in this article refer to percent by weight.) They have excellent cold workability, that is, they can be cold rolled to thin sheet, and despite their relatively high strength, they can readily be shaped into connector components by bending. Being copper alloys, they have adequate electrical conductivity. Their fatigue strengths are high enough to make them suitable for applications where repetitive actions stress the components, as in fasteners, electrical connectors, springs, electrical switches and similar products.
Such components have to retain springiness even if they become warm during operation, such as often happens with electrical switches and connectors. The aim of any connector alloy development program — including those centered on phosphor bronzes — has therefore been to produce a material that has the highest electrical conductivity, highest strength, and best retention of strength at the component operating temperature, combining all of these qualities with ease of fabrication to strip, and ease and consistency of formability to component, all at minimum cost. Quite a tall order, and one which might have been thought too hard to fill until a recent innovation produced a material with improved performance at even lower cost than that of time-tested "veteran" phosphor bronze alloys.
This article describes the steps taken by Olin Corporation metallurgists to create a new connector alloy with an improved combination of the properties mentioned above.
Copper itself is not suitable for use in connectors because (a) it is not springy enough and (b) it loses its strength when held at the operating temperature of most electrical switchgear. Copper, in the form of an alloy, therefore has to be strengthened without undue loss of electrical conductivity.
Phosphorus is added to molten copper to remove oxygen. Phosphorus pentoxide, the product of this deoxidation, is readily removed from the melt during the refining process. Any excess phosphorus goes into solution in the copper, where it provides some strengthening when the copper is cast and worked to sheet. But phosphorus in solution reduces electrical conductivity dramatically. As little as 0.25% phosphorus will reduce conductivity to only 30% IACS, the International Annealed Copper Standard for the pure metal. To make matters worse, too much phosphorus also causes problems during the hot rolling stage of sheet production. The challenge, therefore, is to maintain the amount of phosphorus at an optimal value to remove oxygen, but keep it low enough to enable the material to be worked down to sheet and still retain a relatively high electrical conductivity.
Tin is added to conventional phosphor bronzes to strengthen the alloys. Like phosphorus, tin goes into solution in the molten copper and remains in solution in the solidified alloy. The maximum tin concentration that can be retained in solid solution is about 16%.
Tin in solution produces two conflicting effects. On the positive side, it increases the strength of the copper significantly due to solid-solution strengthening. But the more tin there is in solid solution the lower is the electrical conductivity. Fortunately, the effect of tin on electrical conductivity is less severe than in the case of phosphorus, and 1% of tin only reduces the conductivity to 60% IACS. Again, there has to be a compromise, which depends on the properties required in the final material and the cost of the material. Above 2.5% tin the alloy becomes too expensive to process; below 1.5% tin the alloy lacks strength and the ability to retain strength at its operating temperature.
Hence, conventional phosphor bronzes are a compromise, with optimum additions of phosphorus and tin to give as high a strength as possible coupled with a relatively high electrical conductivity. It should be noted that as the strength of the final rolled sheet material increases, in general so does its ability to retain most of that strength when operated at the temperature of switchgear.
Back to TopStep-by-step Alloy Development
The first step Olin metallurgists took to improve strength was to add iron to the bronze. Iron is not very soluble in copper, and if there is an excess of iron, it precipitates, forming stringers in the material. These stringers make rolling difficult and also affect the formability and final appearance of the sheet; however the advantage of adding iron is that it reduces the grain size of the material both at the cast stage and in the final sheet product. It does this by providing nuclei in the molten copper on which grains can form. Later, during the recrystallization step of the sheet production process, the same iron particles provide a multitude of recrystallization nuclei, which results in fine grain sizes. (The recrystallization step is that during which the cold rolled and therefore work hardened material is softened by heating to a temperature where atoms rearrange themselves to form an ordered structure of well defined crystalline grains.) It is a metallurgical truth that the smaller the grain size of a material, the stronger it is, hence creating smaller grains provides a means to strengthen the alloy.
Besides grain refinement, there are three further benefits resulting from the addition of iron to phosphor bronze. First, iron can be made to combine with some of the phosphorus dissolved in the copper to form iron phosphide, which also appears as tiny particles. This reaction removes phosphorus from solid solution and thereby raises electrical conductivity. Next, the iron phosphide particles provide additional nuclei on which recrystallized grains can grow. This phenomenon keeps grain size small during the recrystallization steps in the production process.
Finally, particles that are present in a material in a sufficiently fine form impart strength to the material by what is called dispersion strengthening. The iron stringers, which break up during the rolling process, and the fine iron phosphide particles produced during annealing both impart strength to the finished sheet by way of this mechanism.
We now have a means of improving the strength of the tin bronze by adding iron, but that, in itself, is not enough. We still have to make the material less costly to produce and use.
Overcoming this challenge came through the innovative substitution of zinc for some of the tin. Zinc is much less costly than tin and can thus reduce the cost of raw materials. Copper will retain as much as 39% zinc in solid solution —three times as much as tin — but zinc reduces the electrical conductivity far less than tin: addition of 1% zinc only reduces conductivity to 90% IACS. The disadvantage is that zinc does not strengthen copper as much as tin does; hence more zinc has to be added to attain the same level of strength. It is therefore a question of balancing the gain in strength against the loss of electrical conductivity.
Fortunately, the amount of zinc that has to be added to maintain strength results in a smaller decrease in electrical conductivity than does the addition of tin. Up to 5% zinc does not increase the strength and only slightly reduces electrical conductivity, but 10% zinc increases strength by about 10% without severely reducing electrical conductivity.
Alloy | Fe | P | Sn | Zn | Cu | Electrical Conductivity %IACS | Tensile Strength Ksi (MPa) | Yield Strength Ksi (MPa) |
---|---|---|---|---|---|---|---|---|
2.2 | 0.06 | 1.8 | Bal | 33 | 99 (682) | 96 (662) | ||
2.2 | 0.06 | 1.8 | 5 | Bal | 29 | 99 (682) | 94 (648) | |
2.2 | 0.06 | 1.8 | 10 | Bal | 25 | 108 (745) | 101 (696) | |
C51000 | 0.033 | 4.27 | Bal | 17 | 102 (703) | 96 (662) | ||
All properties were measured with the alloy in a spring temper after a 70% reduction in thickness by cold working. |
An unexpected side benefit of adding zinc is that it helps reduce grain size in its own right since it appears to enable more of the iron to appear as fine particles.
By looking at the various combinations of iron, zinc, phosphorus and tin additions, optimum quantities of each can be determined to yield an alloy with high strength, relatively high electrical conductivity, good strength retention at working temperatures and low cost. The final nominal composition, now known as UNS C66300, was defined by Olin as:
Zinc | 9.9% |
Tin | 2.2% |
Iron | 1.9% |
Phosphorus | 0.03% |
Copper | Balance |
Commercial Production
The alloy is first cast into slabs by the chill casting process. Slabs are hot rolled in the temperature range 1517-1607F (825 -875C) to reduce their thickness by between 80 and 95%. The surface of the hot rolled material is next machined to remove surface blemishes and oxides.
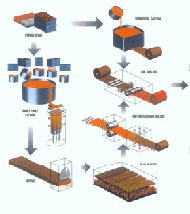
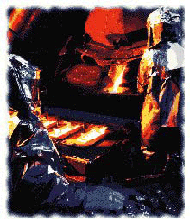
The material next undergoes the first cold rolling operation, reducing the thickness by at least 60%; followed by a recrystallization anneal at 752-1112F (400-600C), typically in a bell shaped annealing furnace containing an inert atmosphere to avoid oxidizing the sheet. It is at this stage that the iron and phosphorus combine to produce iron phosphide particles.
The sheet then undergoes a second cold rolling operation, again reducing the thickness by about 35-45%, and is given a second recrystallization anneal at 752-11121F (400C-600C).
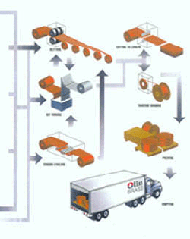
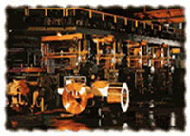
A final temper cold rolling operation is applied to work harden the sheet to the required strength. This is followed by a low-temperature stress relief anneal at 392-572F (200-300C).
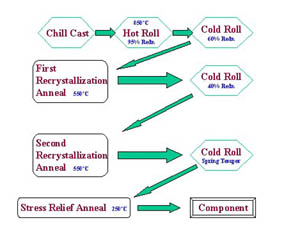
Testing
Samples of the material undergo a bend test to ensure that properties are satisfactory. This test determines the minimum radius the material can be deformed around without cracking. Samples are typically 0.5 in (12.7 mm) wide and are bent around a mandrel of known radius through 90° or 180°. The ratio of minimum bend radius to sheet thickness, MBR/t, that can be used without cracking is taken as a measure of the bendability of the material.
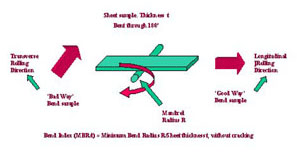
Samples cut with their long axis parallel to the direction of rolling are called "good way" samples and those cut with their long axis at right angles to the rolling direction are called, bad way" samples. (The names describe variations in formability caused by cold rolling.) Tests have shown that the new C66300 alloy is more formable, even in bad way orientations, than veteran alloys C51000, C51100 and C52100.
Alloy | Cu | Fe | P | Sn | Zn |
---|---|---|---|---|---|
C510 | 94.9 | 0.20 | 5.0 | ||
C511 | 95.6 | 0.20 | 4.2 | ||
C521 | 92.0 | 0.19 | 8.0 | ||
C663 | 86.0 | 1.9 | 0.03 | 2.2 | 9.9 |
Resistance of the new material to stress relaxation was determined by preloading a sample to 80% of its yield strength and heating at 257F (125C) for up to 3000 hours. The higher the strength remaining after testing, the better the material. Results of this test suggest that the new alloy exhibits adequate stress relaxation resistance together with excellent electrical conductivity. It can be operated at temperatures up to 257F (125C) and is available in connector tempers with yields strengths up to 100 ksi. It therefore exhibits strength and formability similar to those of alloys C51000, C51100 and C52100 but offers higher electrical conductivity and lower cost.
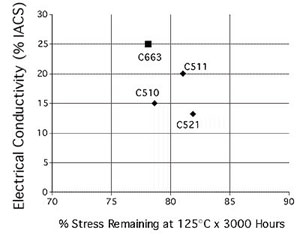
Well, what about cost? Comparison with the alternative materials shows that the new alloy's metal value is lowest, as is its density. In other words, the customer gets more C66300 for less money than with existing alloys.
Alloy | Metal Value $ | Density | Electrical Conductivity % IACS |
---|---|---|---|
C510 | 1.15 | 0.320 | 15.0 |
C511 | 1.12 | 0.320 | 20.0 |
C521 | 1.24 | 0.318 | 13.0 |
C663 | 1.03 | 0.317 | 25.0 |
Based on copper at $1.00/pound |
The material is now finding use as a replacement for phosphor bronze. Applications are expected to arise in spring-type electrical/electronic connectors and terminals, mechanical springs, diaphragms, bellows and contact springs.
Molex Inc, has used C66300 to design and manufacture a Series 2 Intel Xeon processor edgecard connector. This connector has 330 contacts split into three rows for different processor functions.
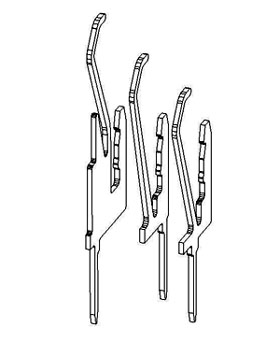
The tallest connector of this type can carry up to 1 A, twice the target figure. The HiSpec® connectors made with the new alloy can withstand 50 connect/disconnect cycles, more than double the previous version. The connector, using the new alloy, is now used by 40 manufacturers to incorporate Xeon processors into their computers.
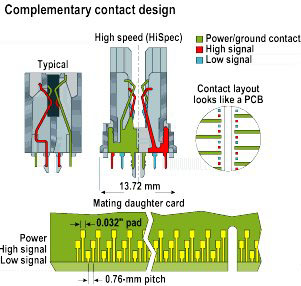
To the metallurgical purist, the new alloy developed by Olin is no longer strictly speaking a phosphor bronze, having been morphed to an iron-modified tin brass. But if the new alloy is cheaper than current alternatives, if it has equivalent or better properties, and if it produces cost-effective components, what's in a name?
Innovations wishes to thank Dr. Derek E. Tyler of the Olin Metal Research Laboratory, New Haven, Connecticut, Dan E. Schuder of Olin Brass, East Alton, Illinois, and Rich Nelson of Molex Inc., Lisle, Illinois, for providing additional information and illustrations. HiSpec is a registered Molex Inc. Trade Name. The patents covered in the article are U.S. 5,882,442, dated 16 March 1999, and U.S. Patent 6,132,528, dated 17 October 2000.
Back to TopAlso in this Issue:
- Phosphor Bronze: Teaching an Old Dog New Tricks
- Copper at the Cutting Edge: The Copper Vapor Laser
- Maitreya Project: Nickel-Aluminum Bronze Selected for World's Largest Statue